Phase Change
Liquid-to-vapor phase change is a ubiquitous phenomenon found in nature and widely used in industry. We are interested in the underlying physics of phase change and their broad applications in power generation, thermal management, seawater desalination, natural gas processing, among others. At the DRL, we are investigating the multiscale and multidisciplinary nature of condensation, boiling and evaporation combining mechanistic modeling with advanced characterizations. By leveraging fundamental understanding along with novel nanoengineered surface designs, we can significantly enhance heat transfer processes for high-efficiency power cycles, desalination devices, and thermal management strategies.
Condensation
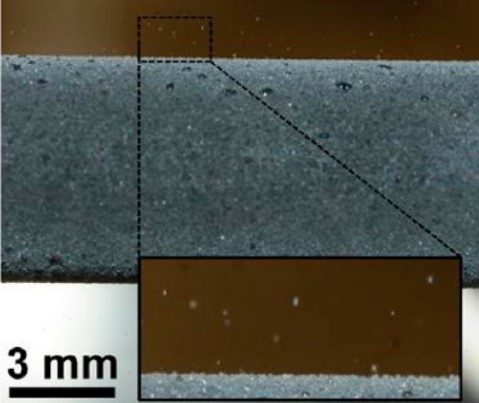
Condensation on nanostructured surfaces
Condensation theory and modeling
Advanced electron imaging for condensation
Boiling
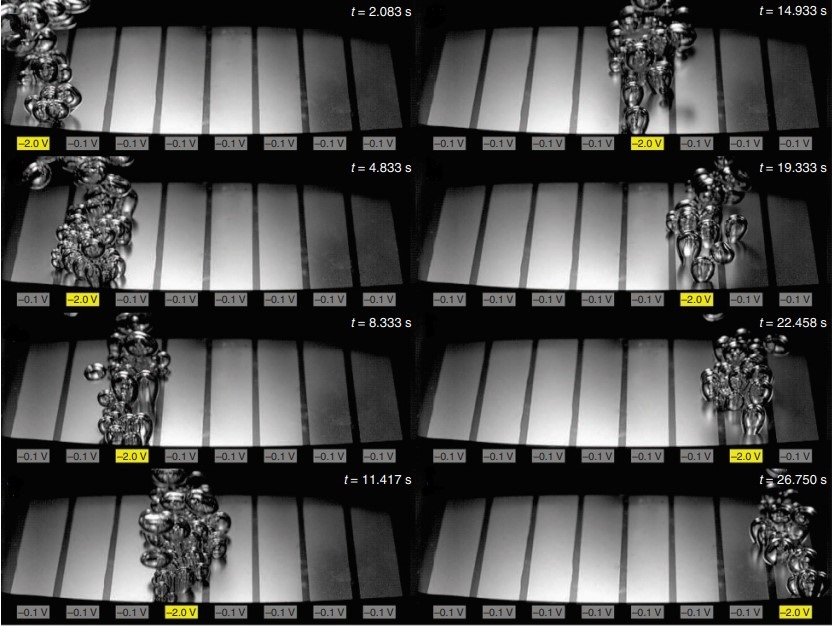
Boiling on structured surfaces
Theoretical framework for boiling
Tuning boiling using charged surfactants
Evaporation
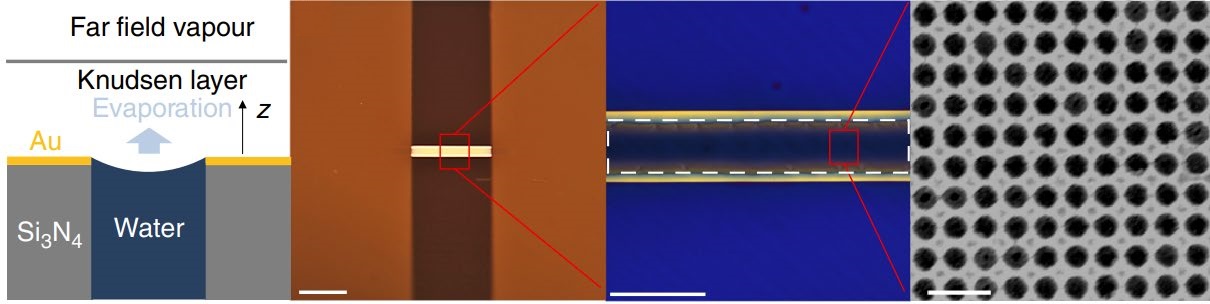
Ultra-thin nanoporous membrane for evaporation
Kinetics of evaporative heat transfer
Recent Progress
Advanced Metrology for Monitoring the Dynamics of Condensation
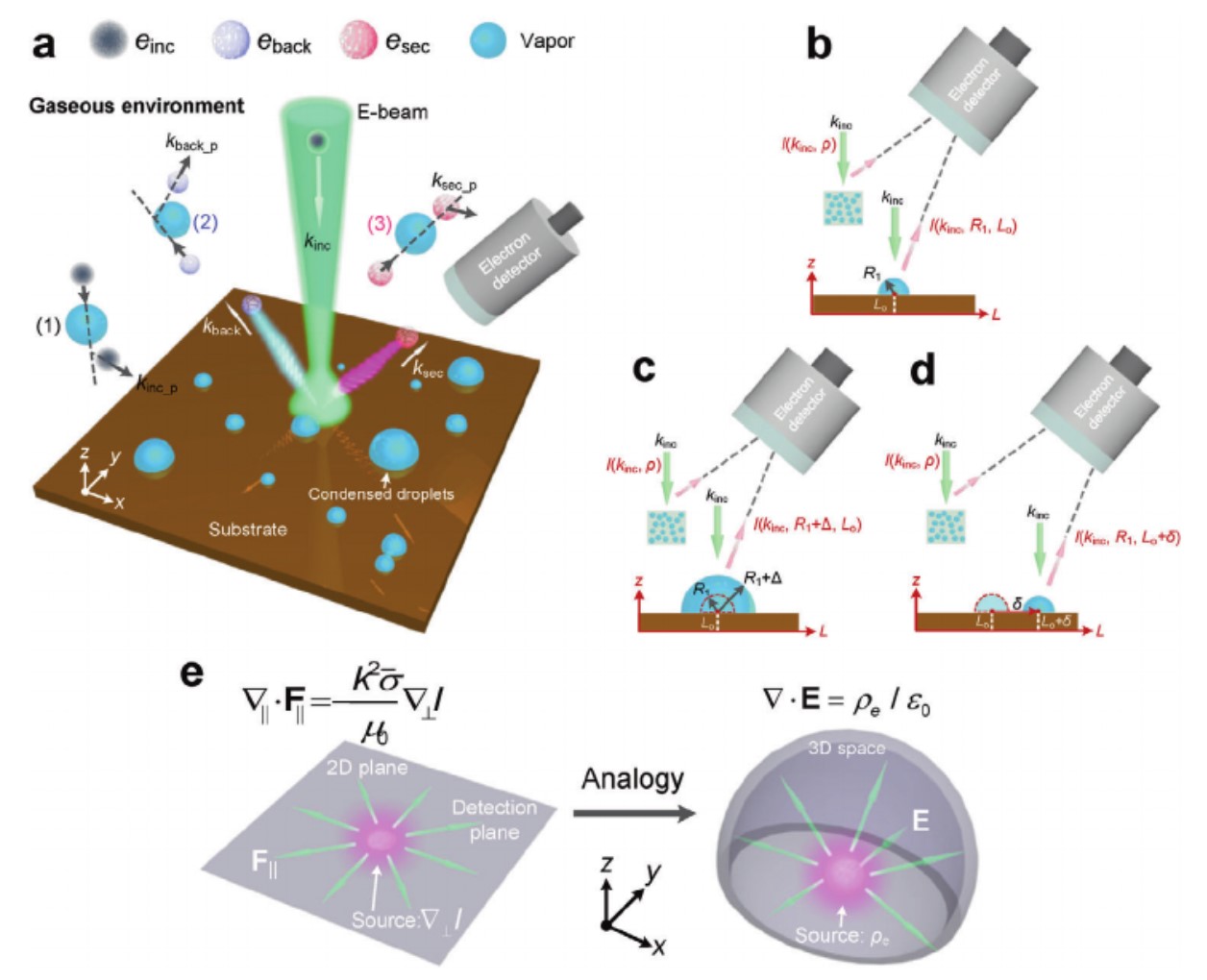
Environmental scanning electron microscopy (ESEM) is a powerful technique that enables imaging of diverse specimens (e.g., biomaterials, chemical materials, nanomaterials) in a hydrated or native state while simultaneously maintaining micro-to-nanoscale resolution. However, it is difficult to achieve high signal-to-noise and artifact-free secondary electron images in a high-pressure gaseous environment due to the intensive electron-gas collisions. In addition, nanotextured substrates can mask the signal from a weakly scattering sample. These drawbacks limit the study of material dynamics under extreme conditions and correspondingly our understanding in many fields. In this work, an imaging framework called Quasi-Newtonian ESEM is proposed, which introduces the concepts of quasi-force and quasi-work by referencing the scattering force in light–matter interactions, to break these barriers without any hardware changes. It is shown that quasi-force is a more fundamental quantity that has a more significant connection with the sample morphology than intensity in the strongly scattering regime. Experimental and theoretical studies on the dynamics of droplet condensation in a high-pressure environment (up to 2500 Pa) successfully demonstrate the effectiveness and robustness of the framework and that the overwhelmed signal of interest in ESEM images can be reconstructed through information stored in the time domain, i.e., frames captured at different moments.
Effect of Hydrocarbon Adsorption on Boiling Heat Transfer
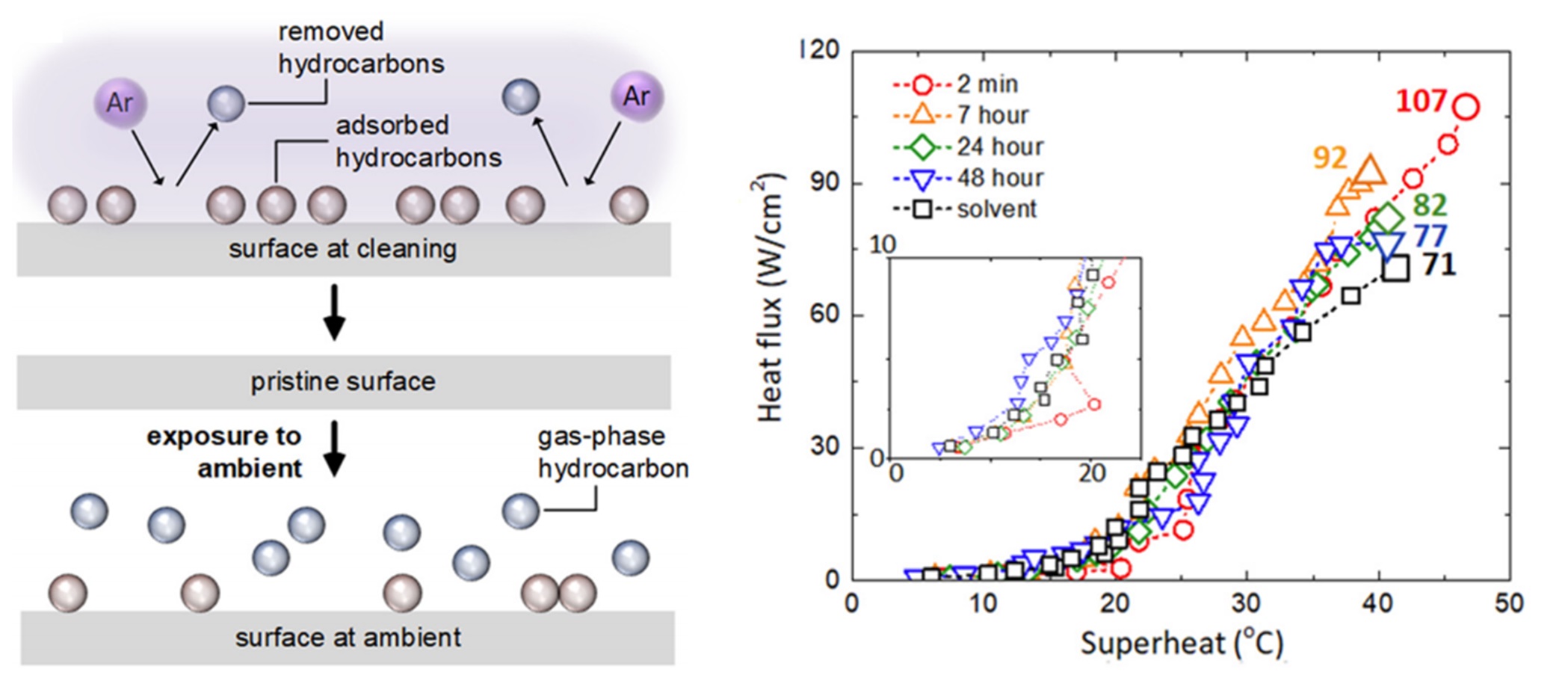
During pool boiling, a significantly high heat flux leads to the transition from nucleate boiling to film boiling, where a vapor film forms over the boiling surface, drastically increasing thermal resistance. This transition at the critical heat flux (CHF) results in an abrupt increase in surface temperature and can lead to catastrophic failure of the boiler. However, reported CHF values vary greatly, even for smooth surfaces of the same material; for example, the CHF values on flat silicon and silicon dioxide surfaces vary across studies by up to 49% and 84%, respectively. Here, we address this discrepancy by accounting for hydrocarbon adsorption on boiling surface. Hydrocarbon adsorption on smooth boiling surfaces decreases surface wettability, hindering the ability to maintain liquid contact with the surface and, thus, lowering the pool boiling CHF. To investigate hydrocarbon adsorption kinetics under ambient conditions and the subsequent effect on CHF, we cleaned flat silicon dioxide samples with argon plasma to remove hydrocarbon contaminants and then exposed them to laboratory air for different periods of time before conducting pool boiling experiments. Pool boiling results along with x-ray photoelectron spectroscopy data showed that the amount of adsorbed hydrocarbon increased with exposure time in air, which resulted in a decrease in wettability and, accordingly, a decrease in CHF. This work has important implications for understanding the spread in CHF values reported in the literature and may serve as a guideline for the preparation of boiling surfaces to achieve consistent experimental results.
Unified Relationship for Evaporation Kinetics
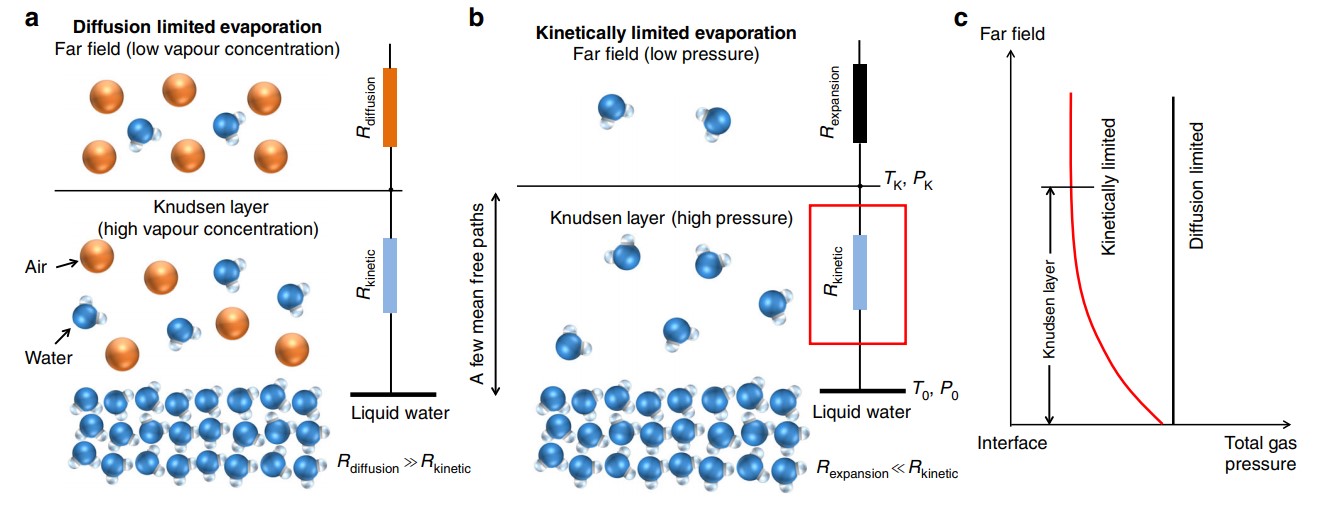
We experimentally realized and elucidated kinetically limited evaporation where the molecular gas dynamics close to the liquid–vapor interface dominates the overall transport. This process fundamentally dictates the performance of various evaporative systems and has received significant theoretical interest. However, experimental studies have been limited due to the difficulty of isolating the interfacial thermal resistance. Here, we overcome this challenge using an ultrathin nanoporous membrane in a pure vapor ambient. We demonstrate a fundamental relationship between the evaporation flux and driving potential in a dimensionless form, which unifies kinetically limited evaporation under different working conditions. We model the nonequilibrium gas kinetics and show good agreement between experiments and theory. Our work provides a general figure of merit for evaporative heat transfer as well as design guidelines for achieving efficient evaporation in applications such as water purification, steam generation, and thermal management.
Related Publications
- H.J. Cho, D.J. Preston, Y. Zhu, E.N. Wang, “Nanoengineered materials for liquid–vapour phase-change heat transfer,” Nature Reviews Materials, 2, 16092, 2016.
- N. Miljkovic, R. Enright, Y. Nam, K. Lopez, N. Dou, J. Sack, E.N. Wang, “Jumping-Droplet-Enhanced Condensation on Scalable Superhydrophobic Nanostructured Surfaces,” Nano Letters, 13, 179, 2013.
- N. Miljkovic, D.J. Preston, R. Enright, E.N. Wang, “Electrostatic Charging of Jumping Droplets on Superhydrophobic Surfaces,” Nature Communications, 4, 2517, 2013.
- D.J. Preston, Z. Lu, Y. Song, Y. Zhao, K.L. Wilke, D.S. Antao, M. Louis, E.N. Wang, “Heat Transfer Enhancement During Water and Hydrocarbon Condensation on Lubricant Infused Surfaces,” Scientific Reports, 8, 540, 2018.
- D.J. Preston, K.L. Wilke, Z. Lu, S.S. Cruz, Y. Zhao, L.L. Becerra, E.N. Wang, “Gravitationally-Driven wicking for enhanced condensation heat transfer,” Langmuir, 34, 4658, 2018.
- K.L. Wilke, D.J. Preston, Z. Lu, E.N. Wang, “Toward Condensation-Resistant Omniphobic Surfaces,” ACS Nano, 12, 11013, 2018.
- L. Zhang, J. Zhu, K.L. Wilke, Z. Xu, L. Zhao, Z. Lu, L.L. Goddard, E.N. Wang, “Enhanced Environmental Scanning Electron Microscopy Using Phase Reconstruction and Its Application in Condensation,” ACS Nano, 13, 1953, 2019.
- L. Zhang, Z. Xu, Z. Lu, J. Du, E.N. Wang, “Size distribution theory for jumping-droplet condensation,” Applied Physics Letters, 114, 1637011, 2019.
- J. Zhu, L. Zhang, X. Li, K.L. Wilke, E.N. Wang, L.L. Goddard, “Quasi‐Newtonian Environmental Scanning Electron Microscopy (QN‐ESEM) for Monitoring Material Dynamics in High‐Pressure Gaseous Environments,” Advanced Science, 7, 2001268, 2020.
- H.J. Cho, J.P. Mizerak, E.N. Wang, “Turning bubbles on and off during boiling using charged surfactants,” Nature Communications, 6, 8599, 2015.
- Y. Zhu, D.S. Antao, K.H. Chu, S. Chen, T.J. Hendricks, T. Zhang, E.N. Wang, “Surface Structure Enhanced Microchannel Flow Boiling,” Journal of Heat Transfer, 138, 091501, 2016.
- Y. Song, L. Zhang, Z. Liu, D.J. Preston, E.N. Wang, “Effects of airborne hydrocarbon adsorption on pool boiling heat transfer,” Applied Physics Letters, 116, 253702, 2020.
- Z. Lu, K.L. Wilke, D.J. Preston, I. Kinefuchi, E. Chang-Davidson, E.N. Wang, “An Ultra-Thin Nanoporous Membrane Evaporator,” Nano Letters, 17, 6217, 2017.
- Z. Lu, I. Kinefuchi, K.L. Wilke, G. Vaartstra, E.N. Wang, “A unified relationship for evaporation kinetics at low Mach numbers,” Nature Communications, 10, 2368, 2019.